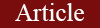
Critical Weed Interference Periods in Quinoa (Chenopodium quinoa Willd.): Yield Loss, Dominant Species, and Management Implications in Andean Agroecosystems
Jorge Merino 1*, Alberto Pedreros 2, Susana Fischer 2 and María D. López 2
1 Instituto Nacional de Investigaciones Agropecuarias (INIAP), Granja Experimental Tumbaco, Pichincha, Ecuador.
2 Universidad de Concepción, Facultad de Agronomía, Av. Vicente Méndez 595, Chillán, Chile; jpedrerosl@udec.cl; sfischer@udec.cl; mlopezb@udec.cl.
*Correspondence: jorge.merino@iniap.gob.ec.
ABSTRACT
Weed competition is a major biotic constraint in quinoa (Chenopodium quinoa Willd.) cultivation, yet the timing and extent of its impact on yield remain poorly understood. This study quantified the effects of weed interference on quinoa yield and identified dominant weed species across two consecutive growing seasons in the Andean region. We evaluated eight weed management treatments (0 to 105 days after emergence, DAE) using a randomized complete block design. Dicotyledonous species dominated the weed community, including Amaranthus hybridus, Ambrosia artemisiifolia, Chenopodium album, and the monocot Echinochloa crus-galli. Weed competition caused a drastic 90% yield reduction, plummeting from 1,352.36 kg ha⁻¹ (weed-free) to 11.55 kg ha⁻¹ when weeds remained until crop maturity. We observed critical yield losses when weeds competed beyond 30 DAE, highlighting the importance of early weed control. We also found significant negative correlations between weed biomass and quinoa growth parameters (plant biomass and grain number; P < 0.05). Echinochloa crus-galli and Polygonum aviculare emerged as key competitors, their dominance shifting with seasonal climatic variations. These findings provide the first empirical basis for integrated weed management in Andean quinoa systems, emphasizing targeted control during early phenological stages to mitigate yield losses. This study advances agroecological strategies for sustainable quinoa production in weed-prone environments.
Keywords: Weed competition; quinoa yield; Andean agriculture; sustainable weed control; dicots vs. monocots; Chenopodium quinoa; weed population; yield loss; critical period of weed interference; agroecological practices; integrated weed management; crop–weed interaction; quinoa cultivation; dryland farming; weed biodiversity
INTRODUCTION
Quinoa (Chenopodium quinoa) is a pseudocereal member of the Amaranthaceae family and has high nutritional value1. It was named the mother grain by the indigenous people of South America, and it has an agricultural history that dates back to approximately 5000-7000 years ago in the Andes region 1,2. This crop can adapt to extreme ecological conditions 1,3. In addition, quinoa resists stress, such as the variation of climates from hot at daylight and cold at night, dry soils with different pH degrees, and exposure to solar radiation 4,5.
Quinoa is an important crop in the Andes of Bolivia, Ecuador, Peru, Argentina, and Chile. Diverse ecotypes, from landraces to modern cultivars obtained through conventional breeding6, can be found. Quinoa has been a low-yield crop in its regions of origin, but as its demand has increased, solutions have been sought to current problems, such as the negative effect of weed populations that emerge alongside the crop cycle. It has been observed that the production of this crop is changing, increasing interspecific competition within weed populations. These changes require new control strategies to reduce the negative effect on yield crops 7,8,9,10. These strategies, such as applying herbicides in specific phenological phases and establishing a critical period of weed interference, should reduce yield losses 8,10. Also, the composition of weeds in the crop should be the starting point for studies. Consequently, the methods for weed management in quinoa are an important factor that directly influences yield; however, there is an absence of information on the composition of the weed populations that grow together with the crop. This study aimed to determine the weed composition of the weed population during crop development and its effect on quinoa yield.
MATERIAL AND METHODS
Plant material and experimental design
"El Nogal" Experimental Station in the Ñuble Region of Chile (36º34' S and 74º06' W) hosted the trials over the course of two consecutive spring seasons (Table 1). Four replicates of a randomized complete block design were employed. The experimental unit measured 4 x 2 m and was composed of four rows of quinoa plants called "Regalona," spaced 0.5 meters apart. Each block contained the eight growing period weed treatments, in which the weeds were allowed to grow for 0, 15, 30, 45, 60, 75, 90, and 105 days after the emergence (DAE) of the crop (Table 2).
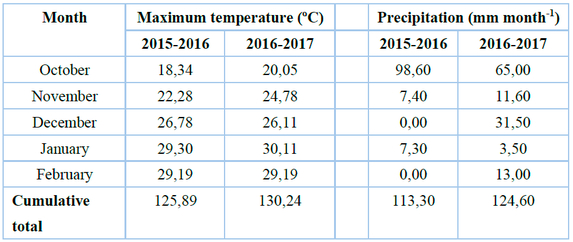
Table 1. Meteorological data of mean temperature and precipitation of the experiment site during the two seasons 2015-2016 and 2016-2017 spring - summer seasons.
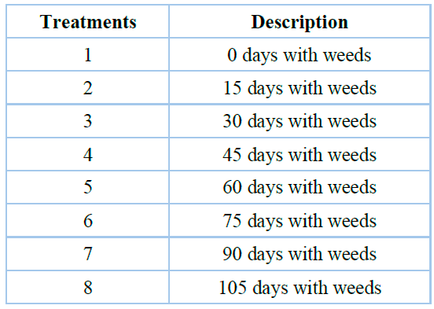
Table 2. Description of treatments in the seasons: spring 2015 - 2016 and spring - summer 2016 - 2017.
Agronomic management
The soil was prepared each season with one moldboard plow pass, two-disc harrow plow passes, and two vibro-cultivator passes. Mid-October saw continuous hand sowing with 0.5 m row spacing and a 12 kg ha-1 seeding rate. Soil analysis revealed that the soil contained 6.8 neutral pH, 8.6 mg accessible N kg-1, 6 mg kg-1 P, and 626.0 mg kg-1 K. P2O5 (100 kg ha-1) and K2O (50 kg ha-1) were evenly administered as fertilizer before seeding, along with N (160 kg ha-1) of which 50% was applied at the four true leaf stage and 50% at the onset of branching. To promote uniform water flow, furrow irrigation was utilized, and hand weed removal was done every two weeks using a hand hoe in compliance with the treatments.
Agronomic variables and weed evaluations
Measurements were taken in two linear meters in the two central rows of each plot. The two central rows of every plot were measured in two linear meters. For the first seven plants from the chosen portion inside the block, measurements were taken of plant height (PH), number of grains per plant, and biomass per plant (g) from the root collar to the apex of the panicle. Every fifteen days (Table 3), the quinoa crop was sampled, and its phenological phase was recorded, with each treatment being linked to the crop phenological stages 11. The two linear meters of the two middle rows of each plot, plus one meter for the boundary on all four sides, were used to calculate the yield per unit area (kg ha-1) variable. After separating the grains from the chaff, the weight of 1000 quinoa seeds was recorded without taking the perigonium into account. This allowed for the determination of the 1000 grains weight (g) variable.
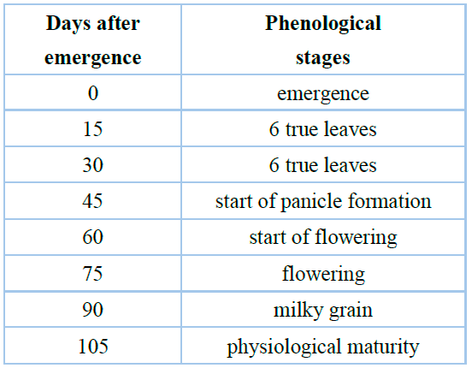
Source: Critical period of weed interference on total polyphenol content in quinoa 12
Table 3. Phenological stages of quinoa "Regalona" in two spring-summer seasons, Ñuble - Chile.
The yield per hectare (kg ha-1), yield per plant (g), plant biomass (g), and number of grains per plant were calculated using the two linear meters of each plot's two middle rows, evaluating the first seven plants of the chosen section.
Quinoa weed population and biomass (dry weight) were assessed using a sampling agreement12. In the middle of each experimental unit's two central rows, a 0.5 × 0.5 m quadrant was drawn. To extract the dry materials, weeds were chopped at soil level, categorized by species, put in paper bags, and oven-dried (FP 115, Binder, Tuttlingen, Germany) for 72 hours at 65 °C.
Statistic analysis
Data was subjected to ANOVA at p < 0.05, and after verifying the assumptions of normal distribution, the Shapiro-Wilk's and Levene's tests were used for homogeneity of variances. Yield data did not show normal distribution and was transformed by the ln (x+1) function. The agronomic traits yield and number of grains per plant were adjusted to an exponential model. The biomass per plant was adjusted to a logistic model in relation to the dry matter of weeds. Means were compared by the LSD test at the 95% significance level. The statistical analysis was performed with the INFOSTAT statistical program13.
RESULTS AND DISCUSSION
A combined experiment analysis compared the results of the variables between the first and second seasons. All the variables' interactions between year and treatment were found to be non-significant (p > 0.05); therefore, the data were aggregated and studied collectively.
Weed population and growth.
Weed plants were not sown, and the population and composition of weeds produced naturally in the experiment site during the two spring-summer seasons at the beginning (15 DAE) and end (105 DAE) of the crop cycle are presented in Table 4, their botanic identification was carried out by using a taxonomic key 14.
The "Other species" classification grouped weed species that did not reach 1% in composition.
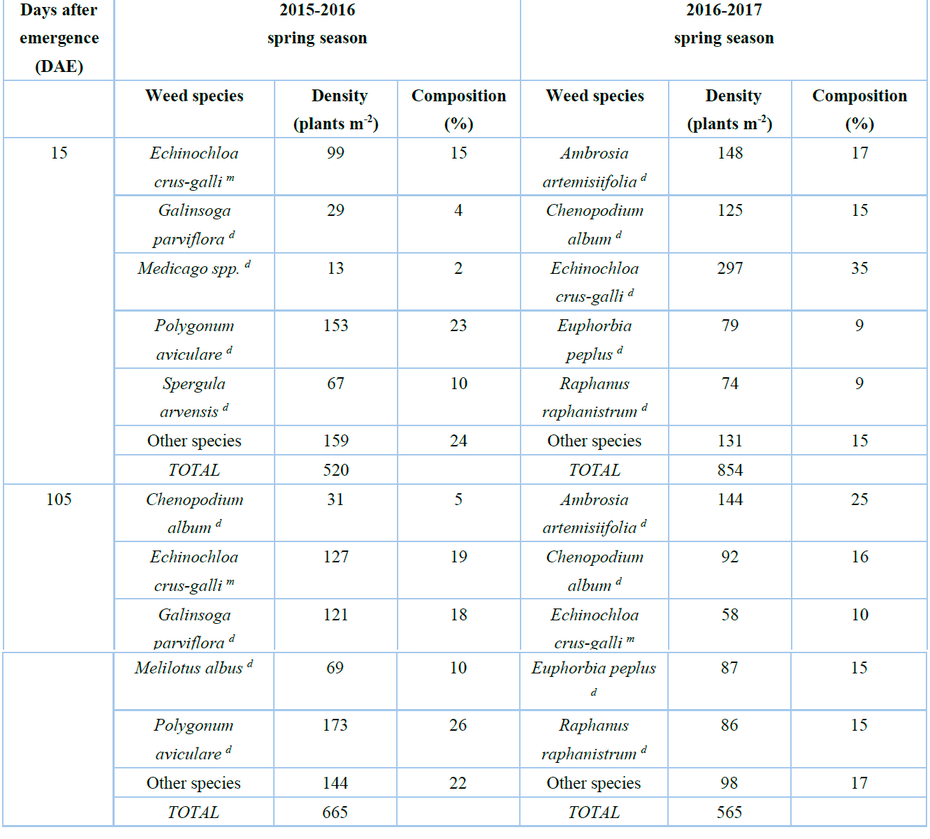
Note: m means monocotyledon, d means dicotyledonous
Table 4. Composition and population of weeds with greater dominance at 15 and 105 DAE during the two seasons.
According to their taxonomy, weeds are classified into two main groups: monocots and dicots15. Monocots are characterized by having a short-lived primary root, which is replaced by a system of adventitious secondary roots, without secondary growth in thickness; their stems have separate, closed vascular bundles, with no secondary thickening; monocot leaves generally have entire blades with parallel veins and are sheathing at the base. Additionally, flowers usually have floral parts in numbers of 3 or multiples of 316. In contrast, dicots have two cotyledons, axile or fasciculate roots, and stems with vascular bundles arranged in open circles. Their leaves can be simple or compound, with a well-differentiated petiole and reticulate venation 17
It was observed that the weed community was composed mostly of dicotyledonous than monocotyledonous species (Poaceae). In general, the main weed species found during the two seasons were Amaranthus hybridus, Ambrosia artimisiifolia, Chenopodium album, Echinochloa crus-galli, Euphorbia peplus, Galinsoga parviflora, Medicago sp., Polygonum aviculare, Raphanus raphanistrum and Spergula arvensis. In the 2015-2016 season, it was observed that Polygonum aviculare was the most common species in all treatments, followed by Echinochloa crus-galli, while in the 2016-2017 season, Echinochloa crus-galli was the most common followed by Chenopidium album. This trend was maintained until 75 DAE; after that, Ambrosia artimisiifolia was the main weed. On the other hand, the population of the quinoa crop remained constant in each evaluation period even though the density of weeds gradually increased to approximately 75 DAE, coinciding with the flowering stage of the quinoa crop, and then decreased near 105 DDE when the crop was in the physiological maturity stage. Although the interference of weeds with the crop did not affect the quinoa population, it did affect the plant's appearance because the quinoa plants were more chlorotic, small, and weak. This implies that maintaining a higher density of weeds until later stages can damage the crop due to intra and interspecific competition for water, light, and nutrients; the shade effect caused by taller weeds is also negative. In pea, an increase in weed density was reported near 60 DAE, and then a decreasing trend was observed, concluding that most weeds emerge up to 60 DAE18.
This difference in dominant species between the first and second seasons may be because Echinochloa crus-galli is an important species that appears in different ecosystems19 because it is a C4 plant, short-day, annual, tropical, and it grows in adverse climates20, 21, adapting to varying ranges of humidity and temperature22. This species prefers high average temperatures (between 28 to 30 °C) because it accumulates more dry matter23; the place where the experiment was carried out had 26 to 29 °C; consequently, the physiological behavior was similar to that reported by Ottis and Talbert 200723. In addition, it produces numerous seeds with a certain level of dormancy, guaranteeing their dispersion and deposit in the soil24, remaining dormant for up to 9 years, and germinating in a staggered manner25.
During the 2015-2016 and 2016-2017 spring - summer seasons, the accumulated precipitation was 113.30 and 124.60 mm year-1, respectively, and the maximum accumulated temperature was 125.89 and 130.24oC respectively. The maximum temperature and precipitation were higher in the 2016-2017 season (Table 4); therefore, it favored the germination of dormant seeds of Echinochloa crus-galli; this species was more abundant (35%) than the rest of the weeds in the experiment site during the first season (2015- 2016). Chenopodium album only appeared at 90 DAE in the second season due to the increase in temperature (0.8 oC) in the second season (2016-2017). Weed seeds are subject to daily temperature fluctuations; thus, the number of seedlings that emerge will depend on the number of seeds that can germinate at soil temperature26. In addition, the proportion of seeds that respond to that temperature depends on the state of dormancy. Therefore, this characteristic hinders the prediction of emergence in field conditions27.
Similar studies have been conducted in other crops; for example, the composition of weeds in three types of corn (Zea mays) was studied during two seasons, finding that Amaranthus retroflexus, Sorghum halapense, and Convolvulus arvensis, were the dominant species in the two seasons28. In potatoes (Solanum tuberosum), Chenopodium album, Amaranthus blitoides, and Amaranthus reflexus were the dominant species in the plots with the highest crop density29. These species are related to our research because they are from the same Chenopodiaceae and Poaceae botanical families.
Comparison with Other Andean Regions
Studies conducted in other Andean countries have reported similar weed dynamics and composition. For example, in the Bolivian Altiplano, Chenopodium album and Amaranthus hybridus have been identified as dominant weed species in quinoa fields, especially under low-input farming systems36. Farmers in Peru's highlands report similar challenges with Echinochloa crus-galli and Polygonum aviculare, particularly under changing climatic conditions that favor early weed emergence38. These patterns support that certain species are regionally adapted and consistently affect quinoa production across diverse Andean agroecosystems7,9. Therefore, regional coordination of weed management strategies, including participatory trials and agroecological approaches, could be essential for improving quinoa productivity and sustainability throughout the Andes.
Grain yield and agronomic variables.
Table 5 shows that the grain yield was affected (p <0.05) by the weed populations that emerged together with the crop and continued to emerge periodically until the quinoa was harvested. It was observed that the time of crop interference with weeds increased from emergence, and yield significantly decreased until 105 days (11.6 kg ha-1). The weed population that remained with the crop until the physiological maturity stage reduced the yield by up to 90%; thus, the intensity and duration of the competition between crop and weeds determines the magnitude of the yield losses10. Weed competes for resources, hosts pests, and decreases grain yield and quality, consequently increasing the cost of production30. Similar studies in other species reported that weeds growing during the entire season reduced the yield of cauliflower31, corn32, pea18, and potato29.
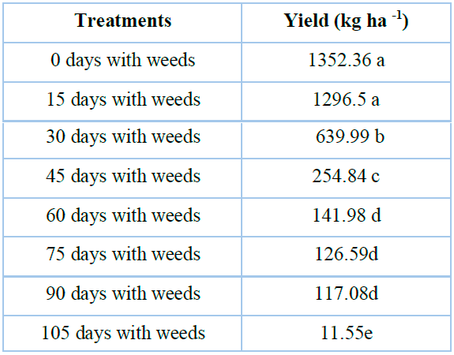
The lower-case letters indicate significant differences between treatments (LSD test P ≤ 0.05)
Table 5. Response of grain yield to periodic populations of weeds in the 'Regalona' quinoa crop.
The biomass per plant was adjusted to a logistic model concerning weed biomass, with a significant coefficient of determination (p <0.05) and an important relationship (R2 = 0.73). Figure 1 shows that the biomass per plant decreased when the weed biomass increased; therefore, the maximum quinoa biomass was obtained in the treatments where weeds were removed (at 0 days with weeds), while the lowest values were obtained in the treatments with high weed biomass (105 days with weeds). On the other hand, the variable number of grains per plant was adjusted to an exponential model concerning weed biomass, with a significant coefficient of determination (p <0.05) and an important relationship (R2 = 0.60). In Figure 2, it was observed that the number of grains per plant decreased as the dry matter of weeds increased; that is, the maximum number of grains per plant was obtained in the treatments that remained without weeds throughout the experiment (0 days with weeds); while the plants with the lowest number of grains were obtained in the treatments that remained with weeds throughout the experiment (105 days with weeds). On the other hand, the weight of the 1000 grains variable was constant (P> 0.05), and the values varied between 3.1 at 15 DAE and 2.9 g at 105 DAE.
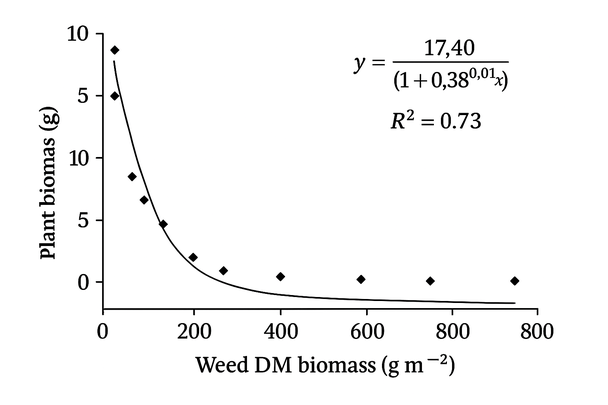
Figure 1. The response between plant and weed biomass in the 'Regalona' quinoa crop, adjusted to a logistic model Y = a/[1+bcX].
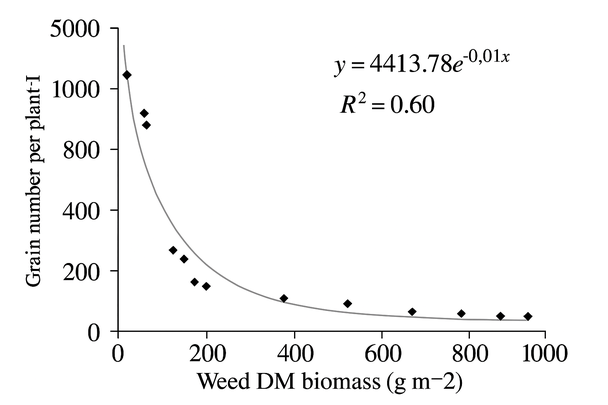
Figure 2. The response between the number of grains and weed biomass in the 'Regalona' quinoa crop was adjusted to an exponential model Y = abX.
The biomass and the number of grains per plant showed their highest values in those treatments that remained free of weeds; this may be due to the shading caused by the taller weeds, which decreased these yield components because of the light availability for photosynthesis and influencing biomass production32. Studies on other crops, such as corn33 and pea18,34, have corroborated that the competition between weeds and crops decreases yield components.
The yield per plant was adjusted to an exponential model about the dry matter of weeds, with a significant coefficient of determination (R2 = 0.72). Figure 3 shows that the yield per plant decreased as the biomass of dry matter of weeds increased; therefore, the maximum yield per plant was obtained in the treatments that remained without weeds throughout the experiment (0 days with weeds), while the lowest yields per plant were obtained in the treatments that presented high weed biomass (105 DAE).
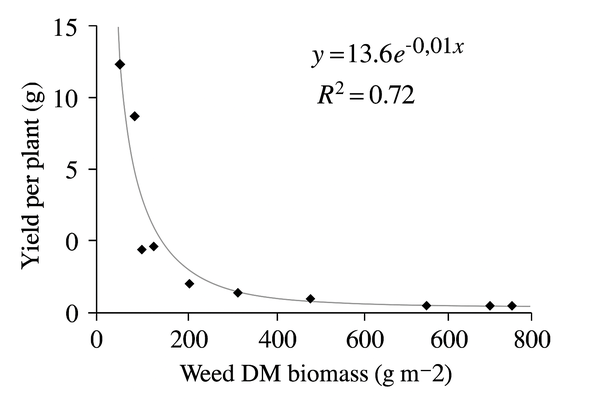
Figure 3. The response between yield per plant and weed biomass in the 'Regalona' quinoa crop, adjusted to an exponential model Y = abX.
High weed biomass directly affects The yield per plant until the advanced stages of crop development due to competition for water, light, and nutrients between the weeds and the crop12. A negative linear relationship was found between weed biomass and yield in rice35. In quinoa, it was found that the treatments that showed the highest weed biomass showed the lowest yield12.
Finally, to understand the dynamics of weeds that inhabit crops during their different growth stages, it is crucial to identify them, as not all species are equally important in the interference they cause to the crop. Each species has the potential to establish itself in a crop or area, and its impact can vary among cultivated plants36. Phytosociological studies analyze the weed community through indices that identify the most significant species to plan management practices and facilitate modifications in control systems37. According to other research38, weeds associated with crops may be linked to the particular characteristics of each region, especially regarding the predominant species. In this study, the composition and presence of weeds were influenced by edaphoclimatic factors; however, monocotyledonous and dicotyledonous species affecting quinoa cultivation were differentiated, and this approach could be replicated in other areas using the same methodology. With the results of this study, along with the determination of the Critical Period of Weed Interference12, an integrated weed management plan for quinoa can be developed, including effective weeding during the determined critical interference period. This will allow producers to reduce production costs and increase crop yield. Future research is suggested to investigate weed composition and the crucial period of interference under different edaphoclimatic conditions to develop integrated management plans adapted to the representative conditions of the crop.
Discussion of Methodological and Ecological Limitations
It is important to acknowledge certain methodological and ecological limitations of this study. Firstly, the experiments were conducted at a single location over two consecutive seasons, which may limit the generalizability of the results to other regions with different soil types, climatic conditions, or weed floras36. The study also focused solely on the 'Regalona' quinoa variety, and weed interference may vary depending on the cultivar's growth habit and competitiveness7. Additionally, although weed species were identified and quantified, the dynamics of seed banks and long-term weed-crop interactions were not analyzed, which are essential components for understanding weed population behavior over time22, 27.
Ecologically, furrow irrigation and manual weed removal may not reflect the practices used by all quinoa producers in the Andean region, particularly in low-input or organic systems 38. Future studies should explore these factors under diverse agroecological settings and consider the inclusion of economic thresholds and environmental impact assessments 9 to strengthen the applicability of integrated weed management recommendations.
CONCLUSIONS
This study provides original evidence on weed populations' seasonal dynamics and species composition affecting quinoa (Chenopodium quinoa) cultivation under Andean conditions. Ten dominant weed species were identified over two consecutive seasons, with a clear shift in dominance from Polygonum aviculare in the first season to Echinochloa crus-galli in the second. This fluctuation, influenced by variations in temperature and precipitation, underscores the relevance of local edaphoclimatic factors in shaping weed community structures. Identifying and tracking such changes represent a novel contribution to understanding weed ecology in quinoa systems.
The results confirmed that the weed community—primarily composed of dicotyledonous species—can cause severe yield losses of up to 90%, particularly when interference extends into the crop's later phenological stages. The study demonstrated significant negative correlations between weed biomass and key agronomic variables such as plant biomass, grain number per plant, and total yield.
These findings provide a scientific basis for developing integrated weed management strategies tailored to quinoa, emphasizing the importance of timely weed control during critical periods. The methodology applied in this study can be replicated in other Andean regions to inform decision-making at both the field and policy levels.
Future research should focus on monitoring long-term weed seed bank dynamics under diverse management practices. Comparative studies on organic versus conventional systems and evaluating non-chemical weed control methods—such as mechanical weeding, mulching, and intercropping—are recommended. Multi-location trials across Andean agroecosystems will be essential to validate critical periods of weed interference and to adapt management practices to local conditions. Furthermore, integrating economic and ecological assessments will enhance these strategies' practical relevance and adoption.
Author Contributions: Conceptualization, Jorge Merino, Alberto Pedreros, Susana Fischer and María D. López; methodology, Alberto Pedreros; investigation Jorge Merino, Alberto Pedreros, Susana Fischer and María D. López; writing—original draft preparation, Jorge Merino; supervision, Alberto Pedreros, Susana Fischer and María D. López. All authors have read and agreed to the published version of the manuscript." Please turn to the CRediT taxonomy for the term explanation. Authorship must be limited to those who have contributed substantially to the work reported.
Funding: This research received external funding from Secretaría de Educación Superior, Ciencia, Tecnología e Innovación (SENESCYT, Ecuador) and the University of Conception (Chile)
Acknowledgments: We thank the Secretaría de Educación Superior, Ciencia, Tecnología e Innovación (SENESCYT, Ecuador) and the Instituto Nacional de Investigaciones Agropecuarias (INIAP, Ecuador) for providing a doctoral scholarship to Jorge Luis Merino Toro. Also, we thank Dr. Jose Luis Zambrano Mendoza for editing this manuscript.
Conflicts of Interest: The authors declare no conflict of interest.
REFERENCES
1. Abugoch, J. 2009. Quinoa (Chenopodium quinoa Willd.): composition chemistry, nutritional, and functional properties. Advances in Food and Nutrition Research 58:1-31.
2. Vega-Gálvez, A., Miranda, M., Vergara, J. Uribe, E., Puente, L. y Martínez, E. 2010. Nutrition facts and functional potential of quinoa (Chenopodium quinoa Willd.), an ancient andean grain: A review. Journal of the Science of Food and Agriculture 90(15):2541-2547.
3. Jacobsen, S. 2003. The worldwide potential for quinoa (Chenopodium quinoa Willd.). Food Reviews International 19:167-177.
4. Gonzalez, J., Gallardo, M., Hilal, M., Rosa, M. y Prado, F. 2009. Physiological responses of quinoa (Chenopodium quinoa Willd.) to drought and waterlogging stresses: Dry matter partitionin. Botanical Studies 50:35-42.
5. Repo-Carrasco-Valencia, R. y Serna, L. 2001. Quinoa (Chenepodium quinoa Willd.) as a source of dietary fiber and other functional components. Ciência e Tecnologia de Alimentos 31(1):225-230.
6. Maughan, P., Bonifacio, A., Coleman, C., Jellen, E., Stevens, M. y Fairbanks, D. 2007. Quinoa (Chenopodium quinoa) C. Kole (Ed.), Pulses, Sugar and Tuber Crops (first ed.), Genome Mapping and Molecular Breeding in Plants, vol. 3, Springer, Berlin pp. 147-158.
7. Chauhan, B., Singh, R., y Mahajan, G. 2012. Ecology and management of weeds under conservation agriculture: a review Crop Protection 38:57-65
8. Chauhan, B. y Opeña, J. 2013. Implications of plant geometry and weed control options in designing a low-seeding seed-drill for dry-seeded rice systems. Field Crops Research 144:225-231.
9. Mhlanga, B., Cheesman, S., Maasdorp, B., Muoni, T., Mabasa, S., Mangosho, E., et al. 2015. Weed community responses to rotations with cover crops in maize-based conservation agriculture systems of Zimbabwe. Crop Protection 69:1-8.
10. Swanton, C., Nkoa, R. y Blackshaw, E. 2015. Experimental methods for crop–weed competition studies. Weed Science 63:2-11.
11. Mujica, A., y Canahua, A. 1989. Fases fenológicas del cultivo de la quinua (Chenopodium quinoa Willd.). p. 23-27. In Curso taller, fenología de cultivos andinos y uso de la información agrometeorológica. Salcedo, 7-10 August. INIAA, EEZA-ILLPA, PICA, PISA, Puno, Perú.
12. Merino, J., Pedreros, A., Fischer, S. and López, María D. 2019. Critical period of weed interference on total polyphenol content in quinoa. Chilean Journal of Agricultural Research 79(3), 405-414.
13. Di Rienzo J.A., Casanoves F., Balzarini M.G., Gonzalez L., Tablada M., y Robledo C.W. InfoStat versión 2017. Grupo InfoStat, Facultad de Ciencias Agrarias, Universidad Nacional de Córdoba, Argentina.
14 Espinoza, N. 1996. Malezas presentes en Chile. Instituto de Investigaciones Agropecuarias, INIA Carillanca. Temuco, Chile. 219 págs.
15. Pereira. E. (2025) Definición de la densidad de impactos en el control de malezas con herbicida total sistémico. Investigación aplicada en campos de las ciencias agrarias y forestales. Universidad Nacional de la Plata Facultad de ciencias agrarias y forestales. 26 pp.
16. Hurrell, J., Bazzano, D., & Delucchi, G. (2006). Biota Rioplatense X. Mocotiledóneas herbáceas nativas y exóticas. Buenos Aires, República Argentina. 322 pp. ISBN: 950-9725-78-1
17. Hurrell, J. (2006). Biota Rioplatense X. Dicotiledoneas herbáceas nativas y exóticas. Buenos Aires. 288 pp. ISBN 950-9725-92-7
18 Singh, M., Kumar, R., Kumar, S., and Kumar, V. 2016. Critical period for weed control in field pea. Legume Research 39(1):86-90.
19 Chauhan, B. 2013. Shade reduces growth and seed production of Echinochloa colona, Echinochloa crus-galli, and Echinochloa glabrescens. Crop Protection 43:241-245
20 Rao, A., Johnson, D., Sivaprasad, B., Ladha, J. y Mortimer, A. 2007. Weed management in direct-seeded rice. Advances in Agronomy 93:153-255.
21 Juliano, L., Casimero, M. y Llewellyn, R. 2010. Multiple herbicide resistance in barnyardgrass (Echinochloa crus-galli) in direct-seeded rice in the Philippines. International Journal of Pest Management 56:299-307.
22 Gallandt, E. 2006. How can we target the weed seedbank?. Weed Science 54:588-596.
23 Ottis, B. y Talbert, R. 2007. Barnyardgrass (Echinochloa crus-galli L.) control and rice density effects on rice yield components. Weed Technology 21:110-118.
24 Clay, S., Kleinjan, J., Clay, D., Forcella, F. y Batchelor, W. 2005. Growth and fecundity of several weed species in corn and soybean. Agronomy journal 97:294-302.
25 Chin, D. 2001. Biology and management of barnyardgrass, red sprangletop and weedy rice. Weed Biology and Management 1:37-41.
26 Batlla, D., 2004. Regulación de los cambios cíclicos en el nivel de dormición de semillas de Polygonum aviculare por efecto de la disponibilidad hídrica y la temperatura del suelo. Un modelo de simulación. Tesis Doctoral. Área Ciencias Agropecuarias. Facultad de Agronomía. Universidad de Buenos Aires. Argentina. Pag. 238.
27 Benech-Arnold, R.. y Sanchez, A. 1995. Modelling weed seed germination. En: Seed development and germination.(Eds J.Kigel, G.Gailili),Pag.545-566.(Marcel Dekker) Inc: New York.
28 Tursun, N., Datta, A., Sami Sakinmaz, M., Kantarci, Z., Knezevic, S., and Singh Chauhan, B. 2016. The critical period for weed control in three corn (Zea mays L.) types. Crop Protection 90:59-65.
29 Ahymadvand, G., Mondani, F., and Golzardi, F. 2009. Effect of crop plant density on critical period of weed competition in potato. Scientia Horticulturae 121:249-254.
30 Zimdahl, R. 2013. Fundamentals of Weed Science. (fourth ed.), Academic Press.
31 Qasem, J. 2009. Weed competition in cauliflower (Brassica oleracea L. var. botrytis) in the Jordan Valley. Scientia Horticulturae 121: 255–259.
33 Safdar, M., Tanveer, A., Khaliq, A., and Maqbool, R. 2016. Critical competition period of parthenium weed (Parthenium hysterophorus L.) in maize. Crop Protection 80:101-107.
32 Vasilakoglou, L., and Dhima, K. 2012. Leafy and semi-leafless field pea competition with winter wild oat as affected by weed density. Field Crop Research 126:130-136
34 Akhter, N., Rahman, M., Hasanuzzaman, M., and Nahar, K. 2009. Plant characters and seed yield of garden pea under different light intensity. American-Eurasian Journal of Agronomy 2(3):152-155.
35 Singh, M., Bhullar, M., Bhagirath S., and Chauhan, B. 2014. The critical period for weed control in dry-seeded rice. Crop Protection 66:80-85.
36. Cruz, D., Silva, G., Oliveira, F., Arcanjo, J. y Alves, J. (2009). Levantamento de plantas daninhas em área rotacionada com as culturas da soja, milho e arroz irrigado no cerrado de Roraima. Agroambiente, 3(1), 58-63. doi:10.18227/1982-8470ragro.v3i1.248.
37. Marqués, P., Silva, M., López, S., Correa, P., Araujo, S., Costa, A. y Muniz, H. (2011). Dinámica de populações e fitossociologia de plantas daninhas no cultivo do feijao-caupi e mandioca no sistema corte e queima com o uso de arado. Planta Daninha, 29(Número Especial), 981-989. doi:10.1590/S0100-83582011000500004.
38 Johanns, O. y Contiero, R. (2006). Efeitos de diferentes perÍodos de controle e convivencia de plantas daninhas com a cultura da mandioca. Ci. Agron., 37(3), 326-331. https://ccarevista.ufc.br/seer/index.php/ccarevista/article/view/175.
Received: September 20, 2024 / Accepted: April 2, 2025 / Published: June 15, 2025
Citation: Merino J, Pedreros A, Fischer S and López MD. Critical Weed Interference Periods in Quinoa (Chenopodium quinoa Willd.): Yield Loss, Dominant Species, and Management Implications in Andean Agroecosystems. Bionatura journal. 2025;2 (2):10. doi: 10.70099/BJ/2025.02.02.10
Additional information Correspondence should be addressed to jorge.merino@iniap.gob.
Peer review information. Bionatura thanks anonymous reviewer(s) for their contribution to the peer review of this work using https://reviewerlocator.webofscience.com/
ISSN. 3020-7886
All articles published by Bionatura Journal are made freely and permanently accessible online immediately upon publication, without subscription charges or registration barriers.
Publisher's Note: Bionatura Journal stays neutral concerning jurisdictional claims in published maps and institutional affiliations.
Copyright: © 2025 by the authors. They were submitted for possible open-access publication under the terms and conditions of the Creative Commons Attribution (CC BY) license (https://creativecommons.org/licenses/by/4.0/).